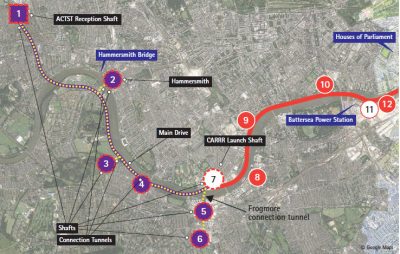
Brought to you by
The design of the Hammersmith connection Tunnel includes a sprayed concrete primary lining and cast in place secondary lining. The waterproofing philosophy adopted by the designer omitted the need for a traditional waterproof membrane between the primary and secondary linings. Instead, a crystallisation agent was specified and incorporated in the primary lining, with the aim of improving the permeability of the section and watertightness of the sprayed concrete joints. The ability of this crystallization agent to limit water ingress was verified through a programme of pre-construction testing. This testing proved that an admixture dosage of 5kg/m3 was sufficient to satisfy the design. During the construction of the tunnel a number of key innovations were implemented by the project team. These included the use of a fully hydrostatic PLC driven formwork system to cast the tunnel secondary lining. The presentation discussed this innovation plus others, whilst providing a detailed overview of both design and construction aspects of the works.
London’s sewers system was designed in the 1950s by Joseph Bazalgette for four million people and cannot accommodate the sewage produced by the now nine million people using them. When the system overflows, treated sewage ends up into the river Thames via the Combined Sewer Overflows (CSOs). This happens on average once per week, resulting in 39Mt of raw sewage entering the river every year. The solution to this problem came in the form of a 25km-long, segmentally-lined tunnel, the Thames Tideway tunnel, which extends from Acton in the West through London, to Abbey Mills Pumping station in the East, collecting 34 of the worst offending Combined Sewage Overflows. When the tunnel enters into operation the sewage will make its way to the sewer where it will be stored and directed to Beckton Sewage Treatment Work for safe treatment.
Given the scale of the scheme, the tunnel was split into three contracts: west, central and east. The East Contract (C405) was awarded to the BAM Nuttall, Morgan Sindall, Balfour Beatty Joint Venture (BMB) in September 2015. It was procured as a NEC Option C single phase design and build contract. BMB used three designers for the contract; Morgan Sindall Engineering Solutions (MSES), for the temporary and permanent works for shafts and the tunnel, Arup-Atkins joint venture, designing the civil structures and Tony Gee and partners for the Marine structures.
The scope of C405 (See Figure 1) includes:
- Seven shafts most of which have been designed and constructed using SCL linings, five of them now complete.
- The main tunnel drive, which is 6.9km-long single bore and 7.1m internal diameter. The drive starts in Carnwath Road and goes west towards Acton Storm Tanks. The TBM, Rachel, has excavated so far 450 rings and about 70,000t of London Clay from beneath the river.
- Four small connection tunnels, being constructed with a range of tunnelling techniques including mechanised tunnelling, pipejacking and SCL.
Hammersmith Pumping Station
How well do you really know your competitors?
Access the most comprehensive Company Profiles on the market, powered by GlobalData. Save hours of research. Gain competitive edge.
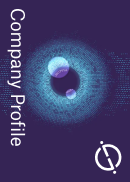
Thank you!
Your download email will arrive shortly
Not ready to buy yet? Download a free sample
We are confident about the unique quality of our Company Profiles. However, we want you to make the most beneficial decision for your business, so we offer a free sample that you can download by submitting the below form
By GlobalDataThe purpose of the works at Hammersmith are to transfer the flows from the pumping station into the main tunnel, which is achieved through and interception chamber, a connection culvert, a drop shaft and a connection tunnel (See Figure 2).
The works in the Hammersmith Pumping Station are taking place in close proximity to existing sewage whilst the station is kept live at all times, as any interruption could result in catastrophic flooding of West London.
The site was handed over to the contracting JV in the summer of 2016 and works have been going on since. The site is surrounded by key residential receptors and sensitive assets such as Frank Banfield Park to the North and residents in the South, West and East. The Interception Chamber is being constructed directly adjacent to the Inlet Channel and works are progressing well with over 4,000t of material having been excavated and the construction of the internal structures has just commenced. Once completed, the Interception Chamber will house the penstock gates which will control the flows into the new Tideway scheme.
The Connection Tunnel, which is 300m long, joins the main tunnel and passes under a number of key assets including the river wall and two blocks of luxury flats with an average value of GBP 1.4M (USD 1.82M).
Geological conditions
The initial site investigation carried out by Thames Tideway comprised only a few boreholes and then BMB undertook further site investigation to confirm the conditions indicated in the Geotechnical Baseline Report (GBR). The geology comprises Made Ground overlaying River Terrace Deposits, the London Clay formation and the Lambeth Group. The connection tunnel is situated in the middle of the London Clay formation with a cover to the Lambeth Group of 19m and with 20m cover of London Clay above the tunnel to the Terrace Gravels. The piezometric level was recorded at 100m above tunnel datum but for design purposes, a level of 105m above tunnel datum was assumed, which corresponds with the ground level.
Design
The client’s reference design indicated the key requirements for the system at Hammersmith. This included a 35m-deep 11m-internal diameter shaft in which a stainless steel, 3.5m-internal diameter vortex tube is constructed.
In the vortex tube the sewage is spiralling from the top of the shaft to the bottom, avoiding in this way a fall which would damage the structure. This has been confirmed by computational fluid dynamics and real scale tests with a model that is 1/10 of the real size of the shaft. The real scale tests indicated that a high quantity of air is generated at the bottom of the vortex tube and therefore it is important that this air is released so that it does not cause any damage in the future. This is carried out through the deaeration chamber and the vent pipe. The deaeration chamber is a 48.1m-long, 5.1m-internal diameter tunnel and the vent pipe is a 1.4m-internal diameter ventilation duct. At the end of the deaeration chamber there is a flow restrictor that holds up the sewage just long enough for the air to rise to the top and travel to the surface through the vent pipe. At the end of the deaeration chamber, a 250m-long, 4m-internal-diameter connection tunnel takes the sewage to the main tunnel. The shaft and tunnels required primary and secondary linings separated by a waterproofing membrane to comply with the strict watertightness requirements. The contracting JV proposed an innovation to remove this waterproofing membrane.
In terms of the water ingress and egress requirements, the maximum allowable is 0.1 l/m2/day on average or 0.2 l/m2/ day over any 10m length of tunnel or 10m depth of shaft. It also required that the water ingress or egress is limited to damp patches defined as a discolouration of part of the surface of the lining, moist to touch but with no visible movement or a film of water across a surface. This statement is open to interpretation and it has been a subject of contention.
The Hammersmith design started in September 2015. The construction of the shaft primary lining finished in December 2017 and for the tunnels, the primary and secondary linings were completed in April 2018. The shaft secondary lining is close to being finished and the internal structures are still ongoing.
The shaft primary lining is constructed using steel fibre reinforced shotcrete with 1.2m advances. As the shaft progresses in depth it has an elliptical shape which incorporates the tunnel opening portal. The thickening section around the tunnel opening incorporates traditional bar reinforcement which is also sprayed in. The base slab at the bottom of the shaft is 2.2m thick and designed as a flat top structural dome which resists the long term uplift forces.
The shaft secondary lining, which is up to 800mm in places, contains both steel fibres and bar reinforcement. The traditional bar reinforcement helps to tie up the internal structures and the vortex tube inside of the shaft.
The tunnel primary lining, 350mm thick, contains steel fibre reinforcement and is installed in 1m advances. The tunnel secondary lining (250mm thick) is designed to avoid the need for traditional reinforcement and uses high performance steel fibres. There are two principal design load cases; one which considers the highest external pressures considering the shaft and the tunnel empty and another which takes into account minimal external water pressures when the shaft and tunnels are full, representing a surge event.
The primary lining is installed sequentially (in advances of 1 or 1.2m) and this takes all the ground load and the initial water load. The secondary lining is then installed in a stress free environment. However, over time the primary lining will deform thus activating the secondary lining and resulting in the two linings working together in a combined manner. During operation, the primary lining will always remain in compression and the secondary lining will go into tension in a surge event.
Watertight Shotcrete
The completed lining comprises 75mm steel fibre reinforced initial layer for the immediate ground support and also provides additional protection from sulphate attack from the groundwater. This layer is not considered to be part of the structural thickness of the structure itself. Following the initial layer, the primary lining, with the same mix and also steel fibre reinforced, uses the steel fibres to control the cracking during the installation when bending is expected and provides the only watertightness of the structure, as there is no traditional membrane between the primary and secondary linings.
The secondary lining is cast against the primary lining and is reinforced with Dramix fibres, even though some areas have traditional reinforcement to be able to control the crack widths to below the required 0.2mm.
In order to have a watertight sprayed concrete lining, the design stipulated a bespoke low cement ratio, low permeability SCL mix to which a crystallisation agent was added to enhance its watertightness. The key focus was on the joints such that they were detailed to be at 45° (see Figure 3), clean and free from contamination and with steel fibres exposed. However, to help with any poor workmanship, a staggered joint sequence was specified so that if potential ingress is coming through the joint the water path is extended reducing the chance of water ingress. Secondary additional measures, including reinjectable tubes and waterbars, were specified at critical areas such as around the base slab and around junctions.
The key requirements for the SCL mixed for the project are presented in Table 1.
The key differences observed from the table with respect to traditional SCL are related to the permeability and the use of the crystallisation agent. The permeability of the normal SCL is usually 1×1010 whereas the specified low permeability shotcrete requires a permeability as low as 1×1012 even within the joints. The crystallisation agent reacts with any moisture that travels through the concrete, forming insoluble crystals within the capillaries of the concrete and further reducing its permeability.
The final mix used by the contractor was developed following an extensive testing programme.
The addition of the crystallisation agent affects the early age strength development of the mix and its plastic properties. The strength development required by the design follows the J++ curve which needs a higher and earlier strength gain than the standard J2 curve used in traditional sprayed concrete.
In the initial samples, the addition of the crystallisation agent, and with 7% accelerator, led to the mix not being able to achieve the required strength at the early hours. In terms of the workability, the addition of the crystallisation agent reduced the retention time from four hours down to under two hours and therefore led to a mix which was not very reliable. It was identified that the crystallisation agent was interfering with the superplasticiser and delaying the strength gain of the concrete and therefore by changing the superplasticiser and the accelerator the mix was able to meet and exceed the J++ curve. This was achieved even at low accelerator dosages (between 5.5% and 6.5%). The workability was also improved to a minimum of two hours, which was deemed adequate by the site team.
Trials were carried out on site in July 2017 on numerous samples taken from forty flexural beams and twenty five panels.
The testing on the panels focused principally on the joints in such a manner that the 45° specified joint was formed at the middle of the panel. This was cleaned with high pressure jet wash and left for 24 hours after which the rest of the panel was sprayed. Once the panel was complete and cured for 28 days, cores would be taken through the middle of the joint to test for compressive strength and more importantly permeability.
Two water penetration tests were undertaken on the mix; the first one being a European standard test as required by the Works Information in which the sample is tested for 72 hours under 5 bars. The second test was developed by Morgan Sindall and the sample was tested for 96 hours under 10 bars. This second tests not only measured the water penetration but also the amount of water left in the sample to ascertain the water permeability coefficient.
After six weeks of trials the contractor developed the final mix which complied with the specifications indicated in Table 1 (b).
It should be highlighted that the addition of the crystallisation agent led to a lower workability and also to a higher price of the mix: GBP 60 (USD 78) per m3.
Shaft construction
Shaft sinking took place in early 2018. The shaft was divided in 29 advances of 1.2m. The staggered joint detail that was explained above was constructed from advance four onwards. Excavation of the shaft always followed the same process with mucking of the centre using an excavator and then trimming the 1.8m deep berm. The next step was the preparation of the joint in which this is first inspected to identify any poor SCL or overspray and if any is encountered this is removed with an excavator mounted rockwheel. Following this, the joint is jet washed with high water pressure and inspected for approval. Every joint required the sign off by an engineer with a minimum of five years’ SCL experience.
The application of the SCL was carried out in two layers with good bond between them.
In terms of testing, BMB set up daily workmanship panels with a frequency of one panel per nozzleman per day. The panels were then cored, inspected for workmanship, compaction, voidage and tested for compressive strength.
The shaft was constructed in 15 weeks, achieving an advance rate of 2.4 advances per week (approximately 3m) and used about 1000m3 of sprayed concrete in the process. The maximum settlement measured was 19.1mm against 19mm predicted.
Tunnel Excavation
The tunnel was constructed in 1m advances and therefore required 295 advances in total. The face was excavated using an ICT120 Heading excavator which excavated 15t of spoil in each round. This was transported using track dumpers to the shaft bottom and from there the spoil was lifted to the top using the top crane.
The excavation was carried out using the laser shell method which uses a remote profiling system called the tunnel beamer, and an inclined face 20° to the vertical. This inclined face significantly reduces the risk of injury associated to SCL fallouts.
Probing ahead has been undertaken from the face extending to 16m ahead of the face and at 12m intervals. This probing ahead has confirmed the expected ground conditions prior to excavation.
The tunnel joint was slightly different to the one in the shaft given the constraints of the tunnel diameter and the lining thickness which made it unfeasible to have a large stagger even though there was still a stagger of 100 mm between the initial layer and the primary lining. The joint was built with an angle of 45°, fibres exposed and free of deleterious material. The preparation of the joint took place in a similar manner to the shaft but the process, however, probed to be more challenging as the rockwheel often damaged the leading edge of the joint.
There was a big focus on dust management during the spraying of the invert section and a blow pipe was used to blow away the rebound in the critical trailing edge.
The tunnel was completed in 22 weeks with an average production rate of 14.1m per week.
In terms of settlement on the tunnel the maximum settlement measured was 10mm, which was in line with the design predictions.
Watertightness achieved
Post construction of the SCL linings an ingress check was undertaken. Table 2 indicates the results of the ingress testing carried out in both the shaft and the tunnel.
Despite the reported results as shown in Table 2, there were some damp patches observed. In the shaft, there were two damp patches corresponding to areas where there were missed core repairs, which indicated that water is actually loading the shaft and watertight.
With respect of the tunnel, nine litres of water ingress in 24 hours were measured, which was less than the 434 litres allowed by the specification. However, there were significant number of damp spots without associated running water, 79 No. on the leading edge of the advances, mostly at the invert. The damp spots were injected with resins in accordance with BS EN1504 using the Typex swelling method. Following the injections, further inspections were carried out and the tunnel signed off as watertight.
The contractor believes that the works at Hammersmith show the potential of a watertight primary lining. Figure 4 shows the comparison between the construction programme achieved for the watertight SCL against what the programme would have been like for a traditional SCL built with sprayed waterproofing. Each waterproofing system has its own merits and therefore one waterproofing system is not better than the other, but the method employed in Hammersmith is another option to consider for future projects. The comparison does not take into account any of the costs or the trialling times required before the works started but as it can be observed there is a potential not only for saving time, reducing the number of activities, reducing the number of interfaces and amount of paperwork and times for approvals but also for reducing the number of resources required. In Hammersmith the application of this techniques led to a programme saving and some small cost saving as well. However, it is considered that with future developments there is a strong possibility that the cost saving can be significantly increased with the cost of the mix and the trialling being reduced.
Construction of the Tunnel’s secondary lining
This is one of the many elements at Hammersmith that was a project first. The design required a full round shutter which was delivered bespoke by Kern for these works (see Figure 5). The shutter takes on board the key learnings from the Lee Tunnel and as a consequence the Hammersmith shutter is only 6 m long as opposed to the 30 m constructed in the Lee Tunnel.
The second key learning was to design the shutter for full hydrostatic head so that it can be filled as quickly as we want to. The length together with the speed the shutter can be filled significantly reduces the risk of cold joints, voids or poor fluid of concrete. The shutter also incorporated a number of key innovations such as:
- PLC Automation system. This is the first shutter to have an integrated PLC so that many elements of the shutter were fully controlled by this project computer which enabled key data collection, and operation of the shutter in semi automatic and automatic modes. As an example, when operated in semi automatic mode, the contractor could not drive the shutter forwards with the spud bars retracted and they could not collapse the skin with the legs protracted.
- Use of automatic spud bars which locked themselves into position following any manual movement, allowed quick setting.
Prior to undertaking the works, the site team carried out comprehensive pretrials on the mix development, starting with three ready mix suppliers until they filtered them down to one. That mix was optimised for filling and vibration in order to achieve a good finish in the invert, which was one of the main issues of the casting with shutters in the Lee Tunnel. This optimisation was achieved with the use of a bespoke testing station which used some of the forms from the Lee Tunnel shutter. The testing station was equipped with a number of features like the tunnel shutter including the same vibrators, the same injection ports and the same ceramic coating. Ten trial panels were undertaken and for each of them the Contractor trial different consistencies, different filling methods (submerged filling or top down filling), different vibration settings in terms of frequency, duration and pattern.
The trial panels were cast simulating the same conditions that the in-situ concrete would have, including the pumping of concrete for 300m until reaching the shutter. Once cured, the shutter was struck and the surface of the panel inspected for defects. Cores were taken to validate the compaction and fibre distribution.
The delivery of the shutter took place in March 2019 with 90% of the structure being assembled at surface before the crane lowered it down the shaft. The remaining 10% of the shutter was attached at the pit bottom where the shutter was fully tested before driving it 300m to the end of the tunnel. The shuttering of the secondary lining took place between 7am to 10pm.
The spud bars probed to be a success as they were really easy and quick to set, and the PLC enabled the contractor to programme a pre-determined preload pressure. Also, during pouring, the sensors in the spud bars enabled the contractor to monitor the pressures, which is a useful tool to identify if there is any differential filling within the shutter. Finally, the contracting JV used a robust timber stopend, required to support hydrostatic pressures particularly in the invert of the framework. All the distribution system was made of steel and the contracting JV started off by using 10 out of the 16 available injection ports.
After a few pours they easily identified that the concrete was performing well and self levelling and therefore the number of injection ports was reduced to four. The final filling sequence was to pump submerged from the invert up to axis level and then close the pour with the two shoulders. The 35 m3 of concrete required for every pour took approximately two hours to fill the shutter.
After a slow learning curve with two to three weeks of a pour every other day they achieve consistently a full pour per day all the way through the tunnel. The finished achieved is of the highest quality even for the invert sections. The tunnel is completely dry with no water ingress observed at all.
Conclusion
The removal of the waterproof membrane was an innovation implemented successfully on site by BMB, which has now set a precedence to potentially use watertight sprayed concrete primary linings in future projects.
The primary lining of the shaft was constructed with complete watertightness without any additional work being required. The primary lining of the tunnel, however, due to the dustier environment probed to be more difficult and some injections were required to bring it back to within specification.
The shift pattern adopted, working week days only, was successful and helped to minimise fatigue, achieved high production rates, retained the workforce and rendered high quality workmanship.
The shutter used for the secondary lining construction included several innovations and was designed taking on board all the lessons learnt from the Lee Tunnel experience.
As a consequence, the final finish of the tunnel exceeded expectations.
All of the key learnings and innovations will be taken forward into the main tunnel which is still to be completed.